A case for a decentralized, hub-and-spoke network of pressure swing adsorption plants
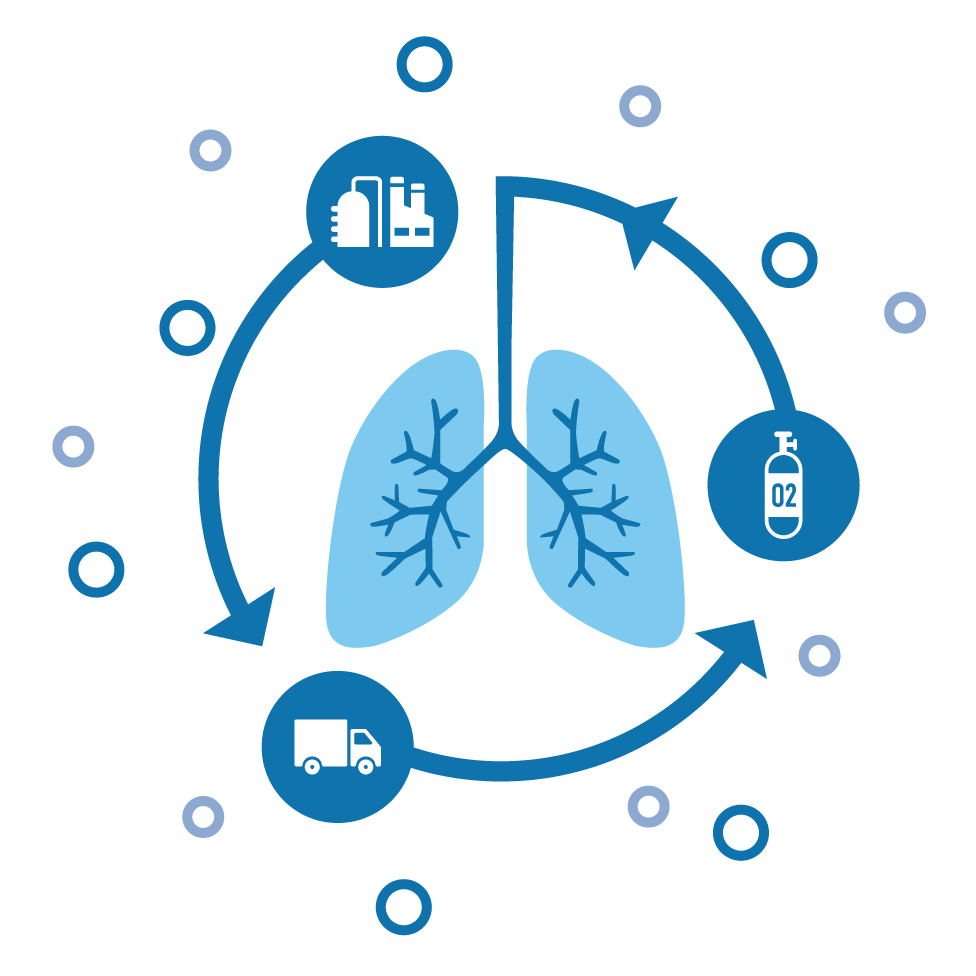
The following is a summary of an analysis published by the Institute for Transformative Technologies (ITT) and Oxygen Hub.
______________________________
Sub-Saharan Africa (SSA) has historically suffered from an acute shortage of medical oxygen. Most countries in SSA have less than 10% of the volume needed to treat high-burden conditions like pneumonia, chronic obstructive pulmonary disease (COPD), and neonatal respiratory syndrome. COVID-19 has both exacerbated and highlighted this oxygen supply gap.
Historically, the most common method of supply in SSA has been in the form of cylinders filled with oxygen gas from cryogenic distillation plants that produce liquid oxygen1 (LOX). Most of these factories were built decades back to serve industrial applications—particularly extractives. To date, they continue to focus heavily on those applications and have served only a fraction of the existing need for medical applications. More recently, smaller pressure swing adsorption (PSA)2 plants have emerged as an alternative to cryogenic plants. However, these systems have often not been adequately maintained, and a sizable portion of them are not currently operational. Unsurprisingly, the existing medical oxygen capacity in SSA has been insufficient to meet the surge needed for COVID-19.
COVID has increased awareness about the importance of oxygen and has generated strong interest from governments, funders, and other influential stakeholders to significantly and sustainably increase access, including to rural areas. It has also sparked a vibrant debate about optimal mechanisms to increase oxygen supply in SSA—especially about the relative merits and disadvantages of PSA vs. LOX as potential solutions at a large scale.
A key finding of our analysis is that—contrary to conventional belief—the per-unit cost3 of oxygen in SSA is similar across the various methods of production. This is because the cost of producing oxygen is only a small portion of the total cost of delivering oxygen to patients, and post-production operations (e.g., cylinder filling, distribution) are virtually the same. Furthermore, many of the LOX facilities in SSA are relatively small, and hence do not benefit from the economies of scale typical of LOX factories around the world.
A second observation is that many SSA countries currently rely on a nontrivial amount of imported oxygen, suggesting that domestic LOX production capacity enjoys high levels of utilization. Third, the majority of people in SSA live in rural areas, and most patients have access only to primary clinics even for conditions that need oxygen treatment. Finally, adding new LOX production capacity in SSA explicitly for medical use will likely be cost prohibitive.
For individual (or a small number of) tertiary hospitals especially in urban/peri-urban areas, the optimal choice is onsite LOX tanks, vaporized on demand and delivered to patients via piped systems (the norm in high-income countries). These hospitals can serve as “anchor users” and can be used as hubs for distributing oxygen cylinders to local primary/secondary clinics. Such a solution may be appropriate for rural tertiary hospitals as well, but only if the transportation infrastructure allows easy and regular access for large LOX trucks. Otherwise, onsite PSA systems may be more appropriate.
However, to reach a meaningfully large population, including in rural areas, an extensive decentralized oxygen infrastructure will be needed. The two choices for such a decentralized infrastructure are networks of (a) PSA plants that produce oxygen and fill cylinders and (b) LOX bulk storage sites with cylinder filling stations, regularly replenished via trucks from a central production facility.
Since the per-unit costs of PSA and LOX are similar, the choice between PSA and LOX in developing a large decentralized infrastructure should be based on the tradeoff between:
LOX and its (i) reliable availability of large volumes of LOX, often via imports; and (ii) reliability of the logistics of regular LOX deliveries via insulated cryotank trucks across a decentralized system.
Or PSA‘s ability to reliably operate and manage the plants.
There is likely enough surplus LOX capacity across the global production system to meaningfully increase the supply of medical oxygen to SSA. However, LOX is a capital-intensive commodity, and a basic economic tendency of capital-intensive production industries is to maximize the utilization of available capacity. As such, it is unlikely there is enough surplus LOX to solve the problem at the needed scale—unless new capacity is developed.
At the same time, the operational complexity of PSA plants with cylinder filling stations is only incrementally more than that of LOX bulk storage sites with the same type of filling stations. We believe this incremental operational complexity offsets the logistical complexity of managing a large number of regular deliveries by LOX tanks. Importantly, PSA plants will ensure much greater local control over a critical lifesaving commodity.
On the margin, therefore, we believe that a decentralized network consisting largely of PSA plants—incorporating LOX stations based on how much supply can be guaranteed—offers the most promising option for a sustainable, self-reliant, large-scale solution to SSA’s medical oxygen deficit.
1 Cryogenic air separation plants also produce a number of other industrial gases.
2 More recently, a variation of the PSA process known as vacuum swing adsorption (VSA) has also emerged as an high-potential option. For the purposes of this discussion, we use the phase PSA to refer to both PSA and VSA.
3 Per-cylinder cost for oxygen suppliers. Note that market price to buyers of oxygen may not reflect the cost.